Atomic Hydrogen
Atom number one
Hydrogen is the lightest and simplest atom in Nature. Experimental studies of properties of H are always of prime importance because of the possibility to calculate them theoretically from first principles. H has played a key role in revealing and testing fundamental laws of physics. Studies of optical spectra led to Bohr theory of the atom, while the discovery of Lamb shift provided foundations of QED. The H maser served for many decades as the frequency standard.
The best of Quantum Gases
It is well known that all gases condense into liquid and then solidify when their temperature is lowered. The only exceptions are helium and hydrogen. Helium goes to liquid state at 1 bar pressure and temperature of 4.2 K. Atomic hydrogen is so light and has so weak interactions between atoms, that the H gas does not liquiefy at all, even at absolute zero. Due to the smallest mass, gas of atomic hydrogen exhibits quantum behavior at higher temperatures than other alkali atoms. In the quantum regime the spatial delocalization of atoms (thermal de-Broglie wavelength) starts to be comparable to the mean ineratomic distance. The atoms cannot be distiguished any more at certain spatial locations, and the whole gas behave as a huge superatom, described by the same wave function. The transition to such quantum state of matter is called Bose-Einstein Condensation (BEC).
Since the midle of the 20th century hydrogen was considered as the best candidate for reaching BEC. Methods of magnetic trapping and evaporative cooling were first suggested for and tested with atomic hydrogen gas. This ensured rapid progress towards BEC, which was reached in 1995 in the gas of Rubidium, the other, more heavy alkali atom. BEC in hydrogen was demostrated three years later, in 1998 in MIT group of D. Kleppner and T. Greytak. Our group at Turku was the first to demonstrate a formation of the quasi-condensate in two-dimensional gas of H adorbed on the surface of superfluid helium. In our later studies we studied magnons – electron spin-waves in dense atomic hydrogen gas. We found that being the bosons, the magnons undergo a transition similar to the BEC of real atoms. This was denmonstrated in experiments of our group in 2015, for the H gas of compresed to the densities exceeding 10^17 cm^-3.
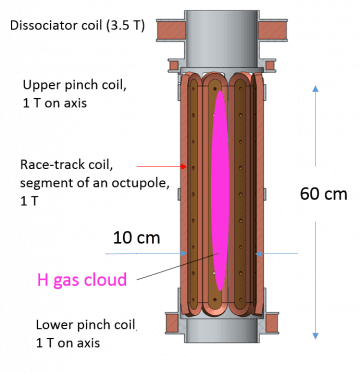
In 2018 a new project was established to study atomic hydrogen gas at extreme condidions of ultra-low energies, when the velocity of atoms is of the order of several cm/s, when they are nearly at rest. Quantum properties of lightest neutral particles: H atoms and neutrons, their interaction with matter, and with the field of gravity are main subjects of this project. Similar experiments are planned with the anti-hydrogen in CERN, and we hope that soon several intriguing tests will
allow direct comparison of fundamental properties of matter and anti-matter.The new project received acronym GRASIAN: GRAvity, Spectroscopiy and Interferometry with Atoms and Neutrons, and was formed originally by a group of researchers from ILL (Grenoble), LKB (Sorbonne, Paris), SMI (Vienna) and our H group in Turku. In 2019 our application to the ERC Synergy call succeeded to the final stage and was very closed to get funding. Currently our group is working on a construction of a large magnetic trap for atomic hydrogen gas . In summer 2020 we completed first tests of the trap, which demonstrated performance sufficient for trapping and cooling H gas. Our trap will have 100 times larger effective volume than it was in MIT treap. We hope that it will allow re-visiting BEC as well as coducting a number of precise spectroscopical experiments. We will focuse on the studies of the H atoms levitating above the surface of supoerfluid helium, in the so-called Gravitational Quantum States (GQS). GQS were first observed by V. Nesvizhevsky in ILL (Grenoble) for neutrons, and similar experiments we plan to perform in Turku with the GRASIAN team.
More detailed information is found on the web page of H group.
Hydrogen atoms inside solids. Quantum diffusion and Supersolid behavior.
Hydrogen atoms can be also stabilized against recombination to molecules, when they are imbedded insilde solid molecular hydrogen and its isotopes: deuterium and tritium. The atoms occypy typically substitutional position in the lattice. Most interesting phenomena which are observed in this system are the quantum diffusion of atoms along the crystal, the possibility of BEC inside the solid crystal. In this project our group conducts research in tight collaboration with the group of V. Khmelenko and D. Lee (Nobel Prize winner in 1996) at Texas A&M University.
Shallow donors in silicon: giant hydrogen-like atoms in condensed media. Qubits for quantum computers?
Silicon crystals with different impurities form a class of semiconductor materials widely used in industrial electronics. If the impurities are from the group V of the periodic table, they are donors of electrons. The donors occupy interstitial positions in the crystalline lattice of silicon. One of the valent lectrons of the donor remains free from the crystalline bonds. It is in the symmetrical s-state, and strongly delocalized due to weak coupling to the donor nucleus. This makes the donor a huge hydrogen-like atom with a large number of the host nuclei inside the highly delocalized donor’s elecgtron cloud. For the silicon of natural isotopical abundunce, 4.8% are 29Si with a nuclear spin of 1/2. Approximately 70 of such nuclei interact with each donor via the super-hyperfine interaction. This makes the donor with the 29Si a very rich system for various magnetic resonance studies. The 29Si nuclear spins can be used as qubits for quantum computing. Recently we demostrated that using high resolution magnetic resonance it is possible to manipulate the nuclkear spins of 29Si occupying definite lattice sites near the donor.